(page 38 in Moving to Higher Ground, © John Englander, 2021)
As identified at the start of Chapter 3, there are three key points that are largely ignored or misunderstood, setting the stage for disaster from future sea level rise.
- Sea level rise this century is unstoppable.
- Science cannot precisely predict the rate of rise.
- The rate of rise can accelerate quickly, possibly abruptly, greatly surprising us.
This note is to give a little more clarity to those three statements and the underlying concepts. To start, let’s recognize that temperature measures how hot something is, but does not describe the amount of heat it contains. For example, it would take far more heat energy to warm a swimming pool 1 degree than to warm a cup of water that same 1 degree. Whereas temperature is measured in degrees, heat energy is most often measured in Calories, as commonly used regarding diets, food intake, and exercise.
Heat can be applied to any substance, whether in the form of heat or radiant energy, like sunlight. That heat or energy is a measurable thing that can be added or removed. Cold may seem similar, but is fundamentally different. Cold is the absence of heat. To make something cooler, we have to take the heat energy out of it and move it somewhere else. You have surely noticed that refrigerators move the heat into the kitchen, just as air conditioners move heat from inside of a house to the outdoors. You can feel the heat coming out of each.
We have warmed the world’s oceans by almost 2 degrees F in the last century or so, but cooling them is not like cooling a building. As refrigerators and air conditioners illustrate, cooling something requires a) considerable energy to power the system to move the heat, and b) a place to put the heat. With those requirements in mind, the naivete to think we can just cool the oceans should become clear.
First, it would take truly gargantuan, almost unimaginable amounts of energy to operate a refrigeration system to cool the ocean. That would add to the problem of global warming, since producing more energy produces more greenhouse gases and more warming, using our current mix of energy sources with heavy reliance on fossil fuels. And even if we could operate the imaginary ocean cooling system without producing greenhouse gases, perhaps from wind, solar, or nuclear fusion, we would still have the problem of where to put the heat. There is no practical way to get it outside the Earth system into the vacuum of outer space.
Small Percentage Changes Significantly Destabilize Systems
Earth continuously receives heat energy from an external source, the sun, and also emits energy to space. For the last 10,000 years, during this turning point of the ice age cycles that brought climate stability, the energy coming in and out of the Earth generally has been in balance. As shown on the illustration below (Figure 5), the incoming solar radiation averaged 342 watts on every square meter (Wm2) of Earth was balanced by the outgoing 107 Wm2 of reflected solar radiation plus 235 Wm2 of outgoing longwave radiation.
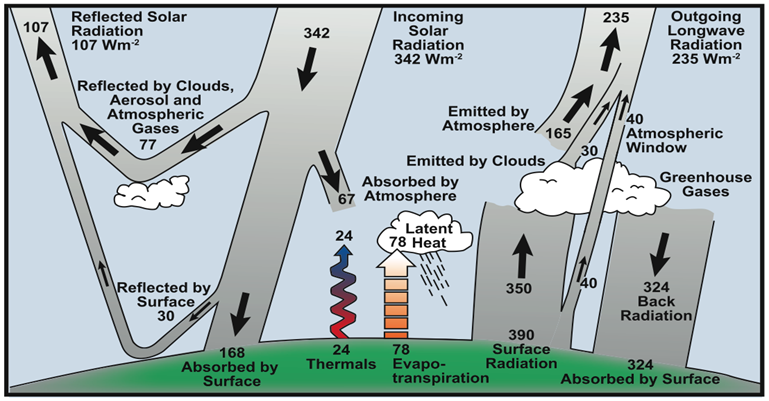
Figure 5: Earth’s temperature depends on the balance of the "energy budget”. If the incoming and outgoing quantities of heat are in balance in a closed system, despite the chaotic weather year to year, the overall planetary temperature stays stable. Increased greenhouse gases are causing an imbalance, gradually raising Earth's heat level (IPCC https://wg1.ipcc.ch/publications/wg1-ar4/faq/wg1_faq-1.1.html).
Now, the greenhouse gases depicted on the right-hand side of the illustration are altering what gets reflected and absorbed. The changes to the composition of our atmosphere have upset the energy balance by roughly 3 Wm2, approximately one percent, causing the planet to warm. Just like we saw with the Milankovitch Cycles in Deeper Dive Note #1, small changes can have dramatic effects.
A good metaphor for the balance of incoming and outgoing energy is heat escaping from a house. If the heating system adds exactly the same amount of heat as escapes, the temperature in the house will stay constant. If you add insulation in the attic or install better insulated windows, you also must lower the heating system output, or the house will get too warm. The continued addition of insulating greenhouse gases to our atmosphere is very similar to adding insulation to your roof.
Referring back to Figure 1, if we were continuing the natural ice age cycles of the last few million years, Earth would be starting on a slow cooling path to another ice age, reaching the coldest temperature about 80,000 years from now. However, our ravenous appetite for energy is adding to atmospheric greenhouse gases. This has caused the Earth system to shift from the initial signs of a cooling phase early last century to what is now clearly a new warming mode. The measured warming force resulting from the greenhouse effect is currently about 100 times greater than the natural cooling force that brings on the ice ages. Like teams pulling on a rope in opposite directions in a tug-of-war, the warming force is 100 times stronger than the cooling force.
Melting Ice Takes Huge Amounts of Energy
In simple terms, to warm a cup of water from say, fifty degrees to sixty degrees, takes the same amount if energy as to warm it from sixty degrees to seventy degrees. Melting ice is something entirely different. It would take eighty times more energy to melt that same cup of ice into water, just above freezing. Thus melting ice consumes a tremendous amount of energy. You can actually prove this with a simple experiment, that is easy to do or just to imagine.
Put a large chunk of ice, or a lot of ice cubes, in a pot of water. Let the water cool for ten minutes or so, to near freezing, which of course is zero Celsius or 32 degrees Fahrenheit. Now put the pot on a stove on high heat and time how long it takes for the last piece of ice to melt. Let’s say, just for example, that it takes 12 minutes to melt that amount of ice, in that pot, on that stove. At the instant when all the ice has melted, the water will not have warmed much because it still had ice in it moments before. As soon as the ice is gone however, things change dramatically. If you leave the heat level on the stove constant, for the same amount of time that it took to melt the ice (12 minutes in my example), the temperature will increase to scalding 80 degrees Celsius or about 175 degrees Fahrenheit. That’s close to boiling temperature, 100 degrees Celsius or 212 degrees Fahrenheit from the same amount of heat, as melting the ice.
Translating this concept to the ocean, the melting ice in the Arctic and Antarctica is consuming a tremendous amount of heat energy just by the process of melting. But as our planet’s ice disappears, there is less ice to absorb the heat energy. The heat energy that is not consumed by melting ice, will cause the water temperature to warm rapidly. In the ocean, the excess does not raise the temperature to eighty degrees, but warms eighty times the volume of sea water.
To summarize, as the ice volume is reduced, the heat energy goes into warming the oceans, which melts more ice, which warms the oceans even faster, which melts the ice even faster, etc. in a “feedback loop” of increasing warming. This warming pace could increase dramatically, possibly, exponentially, affecting the rate of sea level rise. We need to grasp the implications of that uncertainty.
Melting 5% Of The Ice This Century – More or Less
To understand the problem of accurately predicting how high sea level will rise in the next hundred years, it helps to visualize the scale of things. As explained in the second chapter, 98% of the land ice is on top of Antarctica and Greenland. Thus just those two places define the issue rather simply, with Antarctica being about seven times larger that Greenland.
Combined the ice sheets of both would be roughly six million square miles, or sixteen million square kilometers. That’s about twice the size of the continental (contiguous) United States with an ice sheet over a mile high, some six thousand feet. An ice sheet that size does not melt in any linear, smooth predictable manner. The ice sheet in Antarctica covers mountains, valleys, rivers, and volcanoes. Modeling the rate of melting over the coming century is a major challenge to the experts, the glaciologists. In the last few years there has been huge progress improving the models, but it is still a “work in progress” and will be for a very long time.
The latest estimates for SLR this century reflect some great scientific advances, but also the uncertainty described in the book. The latest ice sheet models are very intricate and detailed, but that is not the same as being able to make precise predictions one hundred years in the future, the normal design life of buildings and infrastructure. As with the numerical tools to project pandemics, life expectancy, and earthquakes, they are just models. That is, they are useful tools to understand complex variables and to see how they interact over a range of inputs.
As explained in Chapter 3, models for rising sea level this century now have increased up to ten feet, which would be catastrophic. That would be about five percent of the ice melting. From all of my work on this subject, I think planning for five percent of the ice to melt is about right. Like most estimates however, that should be understood to be plus or minus a few percent. Each percent means another two feet (60 cm) of higher base, global sea level. The implications are huge, in terms of designing and building communities that will be viable and durable.
HEAT UNITS
By definition, 1 Calorie will warm a kilogram of water (2.2 lbs.) 1 degree Celsius (1.8 degrees F). Engineers and scientists may use other heat units, such as BTU’s, joules, or single-calorie units, but the choice of units does not change the concepts described. I use the most common, kilo-calories, or Calorie with a capital letter.